Boost-Phase Interception (BPI) of Ballistic Missiles
Technology Platform, March 9. 2025
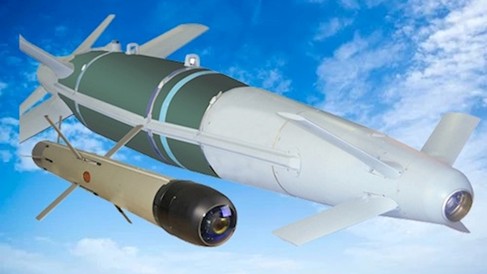
Rephael
Yehoshua Kalisky, Iky Hazan
Intercepting missiles and rockets at the earliest stage of their launch is an offensive action with a distinctly defensive character. Its goal is to prevent direct or collateral damage in the attacked state while inflicting maximum harm on the aggressor. For Israel, this interception method has a compounded advantage due to its small size, the threats posed by Iran and its proxies in both the near and far “ring of fire,” and the high potential for damage from strikes on civilian and military infrastructure. This article reviews the challenges and technological developments in missile and rocket interception, relevant geopolitical aspects, and lessons for the State of Israel.
The State of Israel is the most threatened and attacked country in the world by rocket weaponry of various types, launched at both civilian and military targets. A rocket is defined as a projectile device launched without guidance systems, traveling through the atmosphere toward a land target along a ballistic trajectory. When the rocket includes navigation systems, it is defined as a missile. A missile with an operational range exceeding 100 km is defined as a “ballistic missile.”
Iran and its various proxies—namely, terrorist organizations across the Middle East—as well as the other states within the so-called “axis of evil,” have recognized the advantages inherent in rocket weaponry. Developing and operating these weapons presents a technological challenge requiring extensive multidisciplinary knowledge in physics, chemistry, materials science, thermodynamics, computing, control systems, and electronics, as well as skilled personnel to design and build a rocket weapon system. When the missile is maneuverable, additional expertise in vector-propulsion technology is required—meaning the ability to redirect the missile’s exhaust gases in various directions, thereby altering its flight path toward the intended target.
These technologies are readily available, involving production processes that require accessible raw materials and manufacturing methods that are not especially sophisticated. Operating and maintenance are relatively simple, and their deployment is flexible and straightforward, requiring no specialized professional training. These advantages make the use of rocket weaponry an attractive means of warfare, which is why Israel’s enemies have acquired vast quantities of short- and long-range rockets and missiles to attack both military and civilian targets.
The Challenge Facing Israel
Intercepting ballistic missiles is therefore a paramount technological and security challenge due to the immense damage these weapons can cause, as well as their relative ease of production and operation. Israel is a global leader in developing and implementing missile defense systems through kinetic interception—destroying a missile mid-flight using anti-missile systems such as the Iron Dome, David’s Sling, and Arrow missile system. In the future, as part of Israel’s defense framework, some interceptions will be carried out using directed energy weapons (DEW)—specifically laser weapons—against short-, medium-, and long-range threats.
Israel’s layered missile defense system plays a crucial role in mitigating the damage caused by rocket and missile attacks by intercepting missiles in flight. However, even when threats are neutralized, interceptions over Israeli territory can still cause indirect damage due to falling debris from either the intercepted missile or the interceptor. Additionally, indirect economic damage results from the temporary suspension of activities in targeted areas for periods of time due to air raid sirens and the need to seek shelter.
The most commonly used classification of ballistic missiles is based on their flight range, as shown in the table 1:
Table 1: Classification of Ballistic Missiles by Flight Range
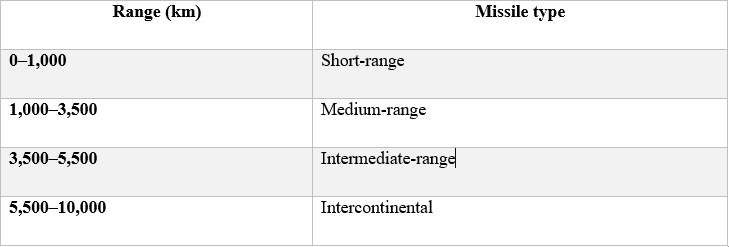
Israel faces two primary threat scenarios: The “belt of fire” threat near its borders, comprising rockets and missiles with ranges of 0–250 km and long-range ballistic missiles launched from Iran and Yemen (and possibly Iraq), with ranges of up to 2,000 km. Addressing this broad spectrum of threats depends on defining the relevant range categories and determining the attack’s point of origin. The timeframe for interception in each operational scenario is set accordingly.
Intercepting a missile as far away from its target as possible has two main advantages. This is why the ideal objective is to neutralize the missile during the boost phase—the period between launch and engine shutdown, when the missile separates from its main body. What makes boost-phase interception advantageous? At this stage, the missile’s speed is still relatively low, it lacks maneuverability, and the thermal and mechanical stresses from the engine operation and fuel combustion make its structure more vulnerable. Once the engine shuts down and the missile separates from its main body, interception becomes more complex, and the potential for collateral damage increases—especially in cases involving nuclear weapons. In any scenario, but especially when dealing with nuclear or other weapons of mass destruction, the primary objective is to contain collateral damage within the aggressor’s territory.
Although concepts for boost-phase interception date back to the 1960s, little progress was made at the time. Interest in the subject resurfaced during the Reagan administration with the launch of the Strategic Defense Initiative (SDI)—popularly known as “Star Wars”—which focused on missile defense, including boost-phase interception. Over the years, the United States introduced various programs to counter the ballistic missile threat at this stage, but apart from limited field tests, none have matured into an operationally proven system.
Much of the information in this article is drawn from a 2022 report by the Center for Strategic and International Studies (CSIS) and a key reference book on the subject.
Boost-Phase Interception
Technological Challenge
The boost phase of a missile typically spans one to five minutes—the window during which the missile’s engine is active and accelerating the missile. At this stage, the missile remains within the atmosphere, its engine is still attached to the main body, its speed is still relatively low, and its maneuverability is limited. Additionally, due to high fuel consumption, the missile’s center of gravity shifts rapidly, creating considerable instability during launch. In principle, a ballistic missile can be intercepted at various stages of its flight (see table 2, figure 1).
Table 2: Stages of a Ballistic Missile’s Flight
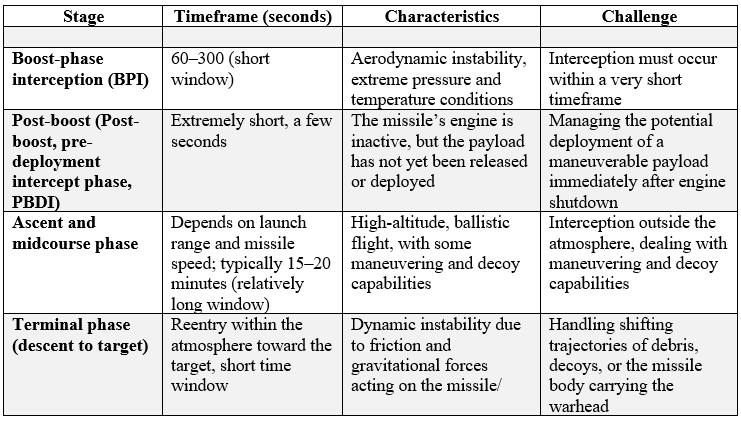
Figure 1. Schematic Representation of a Ballistic Missile’s Flight Phases
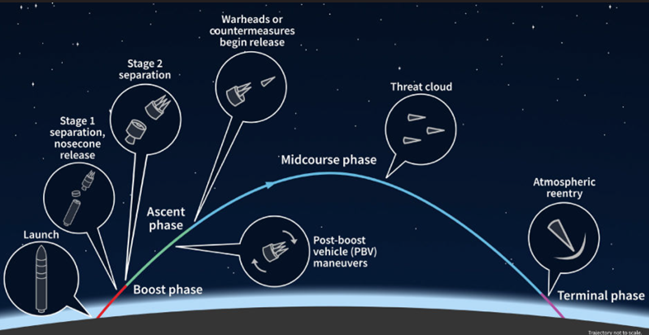
Note: Adapted from Ian Williams and Masao Dahlgren, “Boost-Phase Missile Defense: Interrogating the Assumptions,” 2 (Center for Strategic and International Studies, 2022), https://missilethreat.csis.org/wp-content/uploads/2022/07/220624_Williams_BoostPhase_MissileDefense.pdf
Technological Challenges of Boost-Phase Interception
Currently, the use of BPI technology faces significant technical, economic, and technological limitations. In the near future, BPI may only be feasible through space-based interceptor systems or airborne systems, requiring close proximity to the launch site, highly sensitive and advanced sensor systems with a wide spectral range (i.e., capable of detecting “colors”), rapid image and signal processing, and the capability to launch high-speed interceptors. Successful interception requires a rapid response time, which includes detection and identification, tracking, response planning, the decision to intercept; and hit assessment—including the time needed to confirm whether the interception was successful. Each step in this sequence poses a technological challenge, as summarized in table 3.
Table 3. Sequence of Steps Required for Boost-Phase Interception
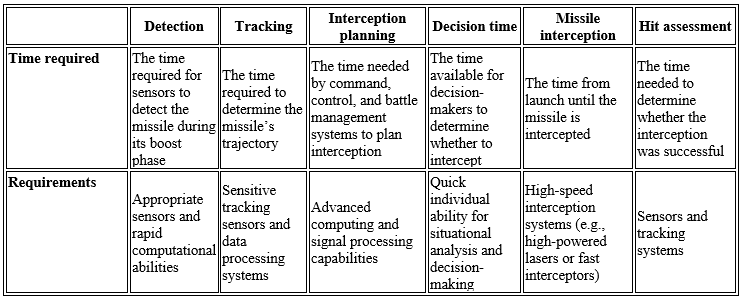
Note: Adapted from Ian Williams and Masao Dahlgren, Boost-Phase Missile Defense: Interrogating the Assumptions (Center for Strategic and International Studies, 2022), https://missilethreat.csis.org/wp-content/uploads/2022/07/220624_Williams_BoostPhase_MissileDefense.pdf
Due to the closer proximity of the airborne interceptor to the target, the kinetic requirements are significantly lower than those of ground-based interceptors. Aircraft can use small, faster interceptors, which, unlike ground-based systems, do not need to accelerate from a stationary position through the dense lower atmosphere. Furthermore, using aircraft offers greater flexibility and a more straightforward integration between the interceptor’s sensor and launch platform, thereby streamlining the interception process.
Since we are focusing on BPI and its advantages over intercepting missiles at later stages, several key points must be emphasized:
During the boost phase of a liquid-fueled ballistic missile, the average temperature in the combustion chamber typically ranges between 2,000 and 3,200°C, while the internal pressure reaches 50 to 200 atmospheres. Physical calculations show that the force exerted per unit area in the combustion chamber during boost depends on the composition of the propellant mixture; for liquid fuel, it can vary from about 3 to 1,000 tons per square meter, whereas with solid fuel, internal pressure may reach several thousand tons per square meter. These extremely high pressures make it easier to damage the missile’s casing—particularly around the engine area, where it experiences extreme stress—potentially causing irreversible failures and even rupturing the missile body during liftoff.
Furthermore, the boost phase precedes the point at which the warhead separates into multiple warheads that then re-enter the atmosphere at high speed, making them more difficult to intercept. Early interception reduces the likelihood that debris or damaged warheads will fall on the target state (for example, Israel) or on friendly third-party territory, thus minimizing collateral damage. A boost-phase interception can also cause the warhead to fall on the attacker’s own territory, potentially causing significant harm there.
The challenges of interception at later stages of a missile’s flight further highlight the benefits of the BPI approach. After the boost phase—during the ascent and midcourse phases until the missile reaches its peak altitude—missiles can employ decoys or other countermeasures (such as dummy targets, signal jamming, radar interference, and flares) and can also perform evasive maneuvers, all of which impair the detection and interception capabilities of air defense systems.
There is a significantly greater challenge in intercepting solid-fueled intercontinental ballistic missiles than liquid-fueled ones, primarily because the preparation and boost phases of solid-fueled missiles are much shorter. Additionally, the boost phase for short- and medium-range ballistic missiles—lasting between one and three minutes—is considerably shorter than that of intercontinental ballistic missiles, which lasts between three and five minutes. The limited timeframe and the lower altitude at which short- and medium-range missiles complete their boost phase make their interception more difficult compared to long-range missiles. Consequently, intercepting solid-fueled missiles and short- to medium-range missiles during the boost phase presents significant challenges and requires the use of faster interceptors positioned closer to the launch site.
Improvements in sensor technologies across a broad spectral range, combined with interception using high-powered laser technologies—potentially deployed in space or on airborne platforms—will enable better interception performance, faster response times, and reduced economic costs. This would make BPI more feasible and sustainable. Moreover, BPI can be integrated into a multi-layered missile defense system, complementing existing interception systems. A multi-layered defense system would allow for the interception of various threats at different stages of missile flight, thereby improving interception success rates and compensating for the gaps in current defense systems.
Technological Developments: Present and Future
Ground and Airborne Interception
Several methods have been proposed for intercepting ballistic missiles during the boost phase, including ground-based kinetic weapons, airborne kinetic weapons, space-based systems, and airborne laser systems. Currently, there is no ground-based system capable of intercepting a ballistic missile during this early phase. The American air defense systems (THAAD, AEGIS, Patriot) and the Israeli systems (David’s Sling, Arrow) are all designed to intercept long-range ballistic missiles either within or beyond the atmosphere.
For ground-based kinetic interception—that is, launching interceptors from the ground—the interceptors must be significantly faster than those launched from airborne platforms. Unlike airborne or space-based interception, ground-based systems do not support laser interception, which requires an extended line of sight to the target—something only achievable at high altitudes. Furthermore, due to the curvature of the Earth and the limited timeframe for interception, there are no ground-based sensors capable of detecting missiles during the boost phase. As a result, ground-based interception requires rapid integration and communication between command and control systems and airborne assets operating within the atmosphere or in space.
A satellite-based detection system equipped with infrared and optical sensors currently exists as part of the American Defense Support Program (DSP). It detects missile launches by remotely sensing the heat emitted by the missile’s engine during launch. The process of detecting the launch, transmitting signals, and processing the information from the moment the missile engine ignites takes an average of 30 seconds. Preparing and launching an interceptor then requires approximately 100 seconds from the moment the alert is received. This means that interception is feasible within the boost phase time frame of a long-range ballistic missile. The prevailing assumption is that the interceptor missile must have a velocity of approximately 7 km per second, allowing it to be stationed 700–1,000 km from the ballistic missile’s launch site.
The development of ground-based interception systems could mitigate security risks and technical limitations associated with airborne interception. It is reasonable to assume (pending further investigation) that this could also reduce the overall costs of maintaining continuous airborne patrols. The potential advantages of ground-based interception could increase the feasibility of BPI for both the United States and Israel, particularly during peacetime. Furthermore, the development of ground-based BPI systems would enable broader cooperation with Middle Eastern allies where such systems could be deployed, as well as the option of placing them on American aircraft carriers or submarines operating in the region.
Developing faster airborne interceptors could significantly enhance BPI capabilities by reducing the time required to complete an interception. To achieve this goal, the interceptor missile must be as lightweight as possible, equipped with highly sensitive optical and thermal sensors to detect the infrared signature of a launching ballistic missile, and designed with advanced aerodynamics and maneuverable propulsion systems to precisely guide the interceptor toward the ballistic missile, particularly near the engine’s “hot” exhaust zone. An example of a technological advancement that could be integrated into a BPI system was introduced at the Paris Air Show in June 2023 by Rafael (see figure 2). This system, the Sky Spear air-to-air missile, is designed with target acquisition, homing, and long-range attack capabilities. As illustrated in figure 2, it is specifically intended to neutralize enemy offensive platforms before they can launch.
Figure 2. Sky Spear Air-to-Air Missile by Rafael
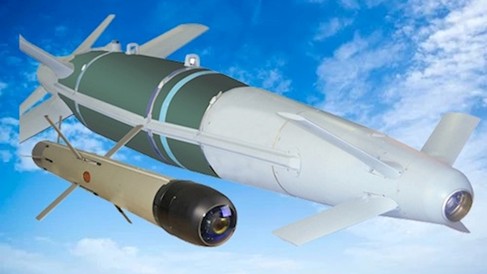
Note: From “Rafael Company Continues to Surprise: It Reveals for the First Time the Rade Missiles,” ICE, June 20, 2023 [in Hebrew], https://www.ice.co.il/finance/news/article/968106?dicbo=v2-qZ5Jv0h
Laser
Unlike kinetic weapons, a laser beam relies on heating the target, requiring it to remain focused on the missile for several seconds to induce structural failure in the missile’s casing. Additionally, lasers significantly reduce interception time, as they can strike a missile almost instantaneously, given that laser beams travel at the speed of light. Since laser weapons do not deplete conventional ammunition, (they are not consumable), their use can substantially lower interception costs and improve the ability to counter multiple simultaneous launches. However, despite these advantages, laser weapons have several limitations. They are restricted to airborne platforms (or potentially space-based systems in the distant future) due to the need for a direct line of sight to the target. Atmospheric interference and optical distortions can significantly reduce the energy density delivered to a specific point on the missile, limiting laser effectiveness.
Chemical lasers, such as the COIL (Chemical Oxygen Iodine Laser), offer advantages due to their long range and high power. However, they also present challenges, including the complexity of transporting and storing the required chemicals, some of which are hazardous, and the limited availability of these materials. These factors restrict their widespread use and require large airborne platforms for deployment. Other laser technologies, such as free-electron lasers (FELs) and ultra-short pulse lasers, are still in the early stages of development and are not yet operationally viable. Similar to chemical lasers, these systems require large and complex infrastructure, preventing their integration into small airborne platforms.
One exception is the Airborne Laser (ABL) system, a COIL laser mounted on a Boeing 747, which operated at an altitude of approximately 12 km. However, it was highly vulnerable to atmospheric disturbances, such as air turbulence and strong winds, necessitating complex optical correction systems to compensate for distortions. Additionally, the system’s performance was poor relative to its cost—estimated at $3 billion—leading to the program’s cancellation in 2012.
Solid-state and fiber lasers (often referred to as “electric lasers”) are more practical for deployment on smaller airborne platforms, such as stealth aircraft or UAVs. However, their lower power output limits their effectiveness for BPI. Their operational range is relatively short and highly dependent on environmental and weather conditions. These lasers are inherently constrained by limited atmospheric transmission, as their laser beams are absorbed by water molecules and various atmospheric gases, scattered by airborne particles, and affected by atmospheric turbulence. Significant progress has been made in developing diode-pumped solid-state and fiber lasers, as well as other laser types. However, despite ongoing technological advancements, no existing laser system appears close to meeting the interception requirements for the boost phase in the near term.
Space-Based Interception
A future breakthrough in developing space-based BPI systems, which the United States may pursue in the long term to counter threats from Russia and China, could significantly improve interception capabilities and benefit Israel as well. Space-based kinetic interception systems offer the advantage of optimal positioning for interception, free from geographical constraints. However, developing such a system is not feasible in the near future due to engineering challenges and the high costs of development and maintenance. Achieving continuous global coverage would require a large constellation of satellites, each with substantial production and launch costs. Additionally, space-based interception systems would be vulnerable to anti-satellite attacks, further reducing their long-term viability.
UAVs
Currently, the airborne platforms most suitable for BPI include fighter jets such as the F-35I stealth aircraft and the F-15, as well as UAVs like the Eitan, Global Hawk, and the Hermes series. Advancements in high-altitude, long-endurance remotely piloted aircraft (HALE RPA)—which can remain airborne for extended periods at high altitudes and lower operational costs—are expected to enhance the feasibility and practicality of using UAVs for boost-phase missile interception. These developments will help reduce production and operational costs, improve interception accuracy and speed, and minimize security risks associated with UAV-based interception.
These improvements are reflected in enhanced aerodynamics of the aircraft, advancements in engine performance and fuel efficiency, and the development of a lighter, more reliable, and smarter structure capable of handling complex challenges. The next generation of American UAVs—set to replace the Global Hawk UAVs scheduled for decommissioning between 2027 and 2029—along with the UAV fleet being developed by the Israeli Air Force and Israel Aerospace Industries as part of the “Storm Clouds” project, including the Spark (“Nitzotz”) UAV, has the potential to significantly enhance performance (see figure 3).
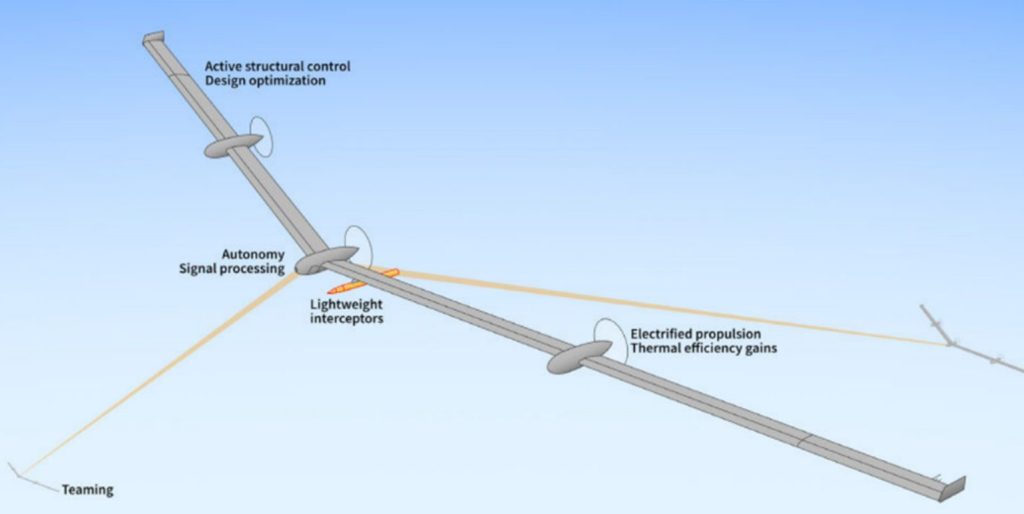
Note: Ian Williams and Masao Dahlgren, “Boost-Phase Missile Defense: Interrogating the Assumptions,” Center for Strategic and International Studies, 2022, https://missilethreat.csis.org/wp-content/uploads/2022/07/220624_Williams_BoostPhase_MissileDefense.pdf
The COIL System Installed on a Zeppelin
A COIL system (Chemical Oxygen-Iodine Laser) mounted on a high-altitude airship (HAA) could serve as a viable method for intercepting ballistic missiles during the boost phase. This system combines the advantages of the COIL laser, which has a long range and high power, with the benefits of using a Zeppelin. Unlike other airborne systems that require more complex logistics to stay near the launch site, the Zeppelin can maintain a fixed position at high altitude, providing continuous protection over a designated area.
Currently, the use of COIL or other chemical lasers, such as those based on hydrogen fluoride-based systems, on airborne platforms is limited due to logistical challenges, including high power consumption for vacuum pumps and water consumption for cooling. Simulations suggest that using a neutral buffer gas at a pressure higher than the surrounding atmosphere could eliminate the need for a vacuum pump in the Zeppelin, allowing it to operate continuously at an altitude of 20 km. At this altitude, atmospheric pressure is low, optical distortions are minimal, and simple optics can be used to strike targets up to 100 km away, with a low interception cost of $100 per shot at a maximum laser output of 1,000 kilowatts. The system’s operational duration could be extended by resupplying chemicals from another Zeppelin or a ship.
However, mounting a COIL system on a Zeppelin comes with several disadvantages and has not yet been tested in operational conditions. The system is vulnerable to weather conditions during takeoff and landing, and its large size and proximity to enemy territory make it susceptible to surface-to-air missile attacks. Additionally, its chemical storage capacity is limited, requiring periodic refueling.
Sensor Development
A key technological advancement for early BPI systems involves improving sensor performance for detecting and tracking missiles during their boost phase. These improvements will significantly reduce the time required to identify a missile and determine its trajectory from the moment of launch. The interception window during the boost phase is extremely short—ranging from 160 to 300 seconds for an intercontinental ballistic missile. Early detection is challenging due to infrared (IR) radiation attenuation, primarily caused by water vapor in the atmosphere; background noise from ground reflections; and the curvature of the Earth, which imposes limitations on early target detection. Advancements in infrared sensor technology will help overcome these challenges, improving interception accuracy and increasing the feasibility of BPI.
Further progress in sensor manufacturing across additional spectral ranges, radar upgrades, and optical component improvements will lower production costs, making these systems more widely accessible. In the short to medium term, ongoing developments are expected to accelerate these improvements.
The integration of new radar technologies, such as gallium nitride (GaN)-based semiconductor radar, could significantly shorten the time needed to detect ballistic missiles. GaN-based radar offers several advantages, including higher voltage tolerance, increased energy efficiency, and improved frequency-switching capabilities. Moreover, it reduces system weight and enhances resolution and range, resulting in more precise and faster tracking capabilities. This also allows for radar integration onto smaller airborne platforms.
Ongoing and future advancements in high-resolution infrared sensors are leading to improved sensitivity, resolution, and efficiency while simultaneously reducing production costs. Shortwave infrared (SWIR) and mid-wave infrared (MWIR) detector arrays provide more precise and sensitive signal detection amid background noise. Additionally, advanced reading technologies using focal plane arrays (FPA) allow for faster data processing and improved target identification and tracking capabilities, even when handling large volumes of data.
These developments, combined with the integration of artificial intelligence (AI), machine learning, and high-resolution image recognition algorithms, will enhance the performance of infrared sensors, enabling missile detection during the boost phase using existing satellites without the need to launch new ones.
Diplomatic Challenges
The diplomatic and technological challenges associated with BPI are closely linked. Successfully intercepting a ballistic threat in its early stage requires positioning airborne or ground-based interception systems at an optimal distance so that the interceptor can strike the missile before engine burnout. This is critical because once the missile accelerates further, it becomes much harder to intercept. However, positioning interception systems in optimal locations often presents diplomatic challenges, especially for countries with limited strategic depth—such as Israel in its confrontation with Iran or the Houthi rebels in Yemen.
For Israel, the lack of strategic depth necessitates rapid interception. However, the significant distance from Iran introduces diplomatic and logistical challenges related to deploying interception systems outside Israel’s borders. BPI is more advantageous for countries located near friendly allies or international waters, particularly those facing a high risk of aerial attack. This scenario requires maintaining constant aerial patrols near enemy territory, which introduces security risks and imposes a substantial economic burden, estimated in the tens of billions of dollars. Consequently, BPI is more feasible during heightened tensions or wartime rather than as a continuous peacetime operation.
A space-based interception system is not discussed here, as its feasibility is still a long-term prospect. However, within a few hundred kilometers of a missile launch site, BPI does not pose a direct threat to strategically deep nations like China or Russia, which can launch missiles from within their own territory.
Regular aerial patrols near enemy borders during peacetime can increase tensions and the risk of escalation. Such activity may be perceived as a provocation or a violation of sovereignty, potentially leading to undesirable security incidents. This creates a gap between the defensive intent of these patrols and their possible misinterpretation as an offensive posture. Additionally, patrolling aircraft face various security threats, including air defense systems, electronic warfare, and adverse weather conditions. The longer the aircraft remain in the air and the more extensive the operations, the greater the exposure to these risks.
Conversely, aerial patrols enhance strike capabilities in enemy territory and serve as a deterrent, discouraging potential attacks for fear of immediate retaliation. Furthermore, the ability to intercept missiles during the boost phase through an Enhanced Forward Presence (eFP) strategy could significantly weaken the effectiveness of enemy ballistic missile use, creating a deterrence-by-punishment effect that could prevent adversaries from launching attacks in the first place.
Given the short reaction time for BPI, decision-makers are left with a narrow window for human decision-making, and real-time international cooperation is challenging. As a result, BPI coordination must be prearranged, requiring each country to act independently in execution. Improvements in sensor technology will shorten missile detection time, allowing for a slightly longer decision-making window.
For Israel, in the case of missile launches from nearby locations (such as Syria and Lebanon), it will have to handle the interception independently. However, long-range launches from Iran or Yemen present a more complex scenario, potentially requiring regional cooperation. BPI is also relevant to East Asia, particularly in countering North Korea’s ballistic missile and nuclear warhead capabilities. The shared need for such systems among US allies (Israel, Japan, South Korea) could serve as a foundation for research and development collaboration.
Operational and Diplomatic Considerations for Regional Application
Iran
Intercepting an Iranian ballistic missile during the boost phase poses significant challenges. Iran’s strategic depth allows it to launch missiles from deep within its territory, making it difficult to position BPI systems near its borders. Effective BPI would require aircraft to regularly patrol close to Iranian airspace.
Figure 4 shows the importance of topography in the operational planning of a boost-phase interceptor missile. The angular orientation of the interceptor’s launch is crucial: a tail-chase intercept requires faster kinematics than a head-on intercept, and sometimes the operational conditions dictate geometry that necessitates very high-speed interceptors. Israel would presumably have difficulty deploying such a system on its own due to economic constraints and technical challenges of operating at long distances, making it dependent on the United States in this case.
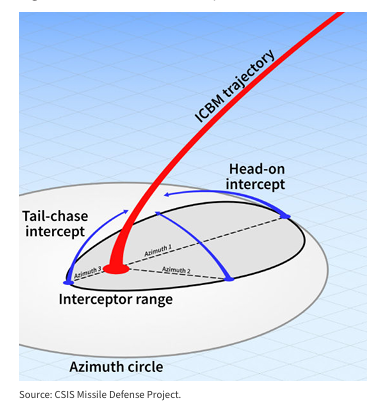
Note: Ian Williams and Masao Dahlgren, “Boost-Phase Missile Defense: Interrogating the Assumptions,” Center for Strategic and International Studies, 2022, https://missilethreat.csis.org/wp-content/uploads/2022/07/220624_Williams_BoostPhase_MissileDefense.pdf
Potential launch bases for interceptor aircraft include US aircraft carriers in the Persian Gulf, along with neighboring countries such as Saudi Arabia, the UAE, and Azerbaijan, where aircraft could operate over the Caspian Sea near Iran. Additional options include aircraft carriers or submarines. However, due to high costs, technical limitations, and significant security risks, continuous BPI operations near Iran are unlikely.
During times of heightened tension, war, or credible intelligence warnings—such as Iran’s missile attacks on Israel on April 14, 2024, and October 1, 2024—the feasibility of BPI using patrolling aircraft becomes more viable.
Houthi Rebels in Yemen
Yemen’s geographic proximity to Saudi Arabia and the Horn of Africa, along with its lack of strategic depth, makes it a more accessible target for BPI. However, the high operational costs of maintaining continuous air patrols near Yemen make routine interception unlikely. Nonetheless, early intelligence warnings could enable targeted BPI missions for specific missile launches.
According to the Lebanese newspaper al-Akhbar, Israel, the UAE, Saudi Arabia, and other nations are reportedly planning military cooperation in the Socotra archipelago, near Yemen. Reports indicate that Israel is constructing a military base on Abd al-Kuri Island within the archipelago, which could serve as a launch site for Israeli interceptor aircraft given early intelligence warnings.
Syria and Lebanon
Due to their proximity to Israel, intercepting missiles from Syria and Lebanon during the boost phase using UAVs and fighter jets is more feasible. This is particularly relevant during wartime or periods of heightened tension when numerous aircraft are already airborne. However, the boost phase for short- and medium-range missiles launched from these locations is very short, posing a significant technological challenge for air-based interception.
If integrated into a multi-layered defense system, BPI could enhance Israel’s ability to counter Hezbollah’s precision missile arsenal and Syria’s missile capabilities alongside the Arrow and David’s Sling systems. Given the uncertain future of the northern front, BPI remains a relevant consideration.
Recommendations and Lessons for Israel
Israel is the most threatened country in the world regarding rockets and missiles of all types. The objective of these threats is to encircle Israel in a “ring or belt of fire,” following the doctrine promoted by Qassem Soleimani, who was commander of Iran’s Islamic Revolutionary Guard Corps, alongside a prolonged war of attrition carried out by non-state actors—Iran’s proxies—whose ultimate goal is the destruction of Israel. To counter this multi-range rocket and missile threat, which endangers civilian and military infrastructure, Israel has deployed multi-layered defense systems. Integrating a BPI system into Israel’s defense network would add an additional protective layer with clear operational advantages. BPI offers several key benefits: Missile debris, engine parts, or remnants of the missile body and warhead would fall within enemy territory, preventing direct and collateral damage to Israeli civilians and infrastructure; early interception within enemy territory eliminates the need to send large civilian populations into protected areas, reducing economic and psychological disruption.
When designing an early interception system, it is essential to assess the future trajectory of an interceptor missile to avoid unnecessary interceptions of missiles that are not targeting populated areas or strategic sites. Reports in the Israeli press indicate that in 1995, Rafael began developing the “Moab” missile for the early interception of rockets and missiles, but beyond reports of certain challenges, there is no information regarding the project’s progress. Given the clear advantages of early interception during the boost phase, it is recommended to consider integrating this capability as part of Israel’s active defense network, which would significantly ease the burden on existing systems. Additionally, the Sky Spear missile could serve as a potential BPI solution. However, its integration would require advanced detection and tracking systems operating across specialized spectral ranges, coupled with high-speed data processing capabilities, since the window of opportunity for BPI is extremely short.
Given the advantages of BPI and the broad range of threats facing Israel, the Ministry of Defense should allocate research funding and resources toward the development of this capability. At the same time, the military and diplomatic aspects of rapidly implementing a BPI system should be carefully considered.